As with many of mankind's monumental discoveries, X-ray
technology was invented completely by accident. In 1895, a
German physicist named Wilhelm Roentgen made the discovery
while experimenting with electron beams in a gas
discharge tube. Roentgen noticed that a fluorescent
screen in his lab started to glow when the electron beam was
turned on. This response in itself wasn't so surprising --
fluorescent material normally glows in reaction to
electromagnetic radiation -- but Roentgen's tube was
surrounded by heavy black cardboard. Roentgen assumed this
would have blocked most of the radiation.
Roentgen
placed various objects between the tube and the screen, and
the screen still glowed. Finally, he put his hand in front of
the tube, and saw the silhouette of his bones projected onto
the fluorescent screen. Immediately after discovering X-rays
themselves, he had discovered their most beneficial
application.
Roentgen's remarkable discovery precipitated one of the
most important medical advancements in human history. X-ray
technology lets doctors see straight through human tissue to
examine broken bones, cavities and swallowed objects with
extraordinary ease. Modified X-ray procedures can be used to
examine softer tissue, such as the lungs, blood
vessels or the intestines.
In this article, we'll find out exactly how X-rays machines
pull off this incredible trick. As it turns out, the basic
process is really very simple.
Go to TOP
What's an X-Ray?
X-rays are basically the
same thing as visible light rays. Both are wavelike forms of
electromagnetic energy carried by particles called
photons. The difference between X-rays and
visible light rays is the energy level of the
individual photons. This is also expressed as the
wavelength of the rays.
Our eyes are
sensitive to the particular wavelength of visible light, but
not to the shorter wavelength of higher energy X-ray waves or
the longer wavelength of the lower energy radio
waves.
Visible light photons and X-ray photons are both produced
by the movement of electrons in atoms.
Electrons occupy different energy levels, or orbitals, around
an atom's nucleus. When an electron drops to a lower orbital,
it needs to release some energy -- it releases the extra
energy in the form of a photon. The energy level of the photon
depends on how far the electron dropped between orbitals.
When a photon collides with another atom, the atom may
absorb the photon's energy by boosting an electron to a
higher level. For this to happen, the energy level of the
photon has to match the energy difference between the
two electron positions. If not, the photon can't shift
electrons between orbitals.
The atoms that make up your body tissue absorb visible
light photons very well. The energy level of the photon fits
with various energy differences between electron positions.
Radio waves don't have enough energy to move electrons between
orbitals in larger atoms, so they pass through most stuff.
X-ray photons also pass through most things, but for the
opposite reason: They have too much energy.
They can, however, knock an electron away from an atom
altogether. Some of the energy from the X-ray photon works to
separate the electron from the atom, and the rest sends the
electron flying through space. A larger atom is more likely to
absorb an X-ray photon in this way, because larger atoms have
greater energy differences between orbitals -- the energy
level more closely matches the energy of the photon. Smaller
atoms, where the electron orbitals are separated by relatively
low jumps in energy, are less likely to absorb X-ray photons.
The soft tissue in your body is composed of smaller atoms,
and so does not absorb X-ray photons particularly well. The
calcium atoms that make up your bones are much larger, so they
are better at absorbing X-ray photons.
In the next section, we'll see how X-ray machines put this
effect to work.
Other X-Ray
UsesThe most important
contributions of X-ray technology have been in the world
of medicine, but X-rays have played a crucial role in a
number of other areas as well. X-rays have been pivotal
in research involving quantum mechanics theory,
crystallography and cosmology. In the industrial world,
X-ray scanners are often used to detect minute flaws in
heavy metal equipment. And X-ray scanners have become
standard equipment in airport
security, of course.
|
Go to TOP
The X-Ray Machine
The heart of an X-ray
machine is an electrode pair -- a cathode and an anode
-- that sits inside a glass vacuum tube. The cathode is
a heated filament, like you might find in an older fluorescent
lamp. The machine passes current through the filament,
heating it up. The heat sputters electrons off of the filament
surface. The positively-charged anode, a flat disc made of
tungsten, draws the electrons across the tube.
The voltage difference between the cathode and anode is
extremely high, so the electrons fly through the tube with a
great deal of force. When a speeding electron collides with a
tungsten atom, it knocks loose an electron in one of the
atom's lower orbitals. An electron in a higher orbital
immediately falls to the lower energy level, releasing its
extra energy in the form of a photon. It's a big drop, so the
photon has a high energy level -- it is an X-ray photon.
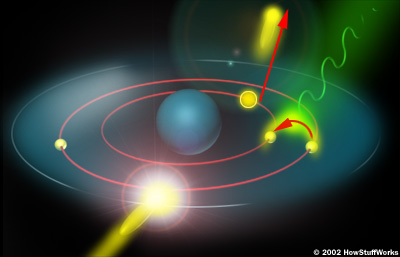 The free electron collides with the tungsten
atom, knocking an electron out of a lower orbital. A
higher orbital electron fills the empty position,
releasing its excess energy as a
photon.
|
Free electrons can also generate photons without hitting an
atom. An atom's nucleus may attract a speeding electron just
enough to alter its course. Like a comet whipping around the sun,
the electron slows down and changes direction as it speeds
past the atom. This "braking" action causes the electron to
emit excess energy in the form of an X-ray photon.
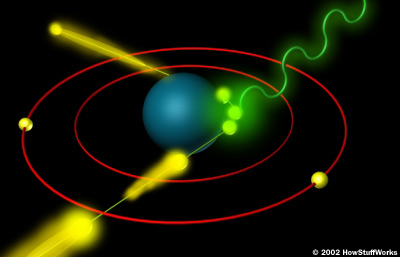 The free electron is attracted to the
tungsten atom nucleus. As the electron speeds past, the
nucleus alters its course. The electron loses energy,
which it releases as an X-ray
photon.
|
The high-impact collisions involved in X-ray production
generate a lot of heat. A motor
rotates the anode to keep it from melting (the electron beam
isn't always focused on the same area). A cool oil bath
surrounding the envelope also absorbs heat.
The entire mechanism is surrounded by a thick lead shield.
This keeps the X-rays from escaping in all directions. A small
window in the shield lets some of the X-ray photons escape in
a narrow beam. The beam passes through a series of filters on
its way to the patient.
A camera on the other side of the patient records the
pattern of X-ray light that passes all the way through the
patient's body. The X-ray camera uses the same film technology
as an ordinary
camera, but X-ray light sets off the chemical reaction
instead of visible light.
Generally, doctors keep the film image as a
negative. That is, the areas that are exposed to more
light appear darker and the areas that are exposed to less
light appear lighter. Hard material, such as bone, appears
white, and softer material appears black or gray. Doctors can
bring different materials into focus by varying the intensity
of the X-ray beam.
Contrast
MediaIn a normal X-ray
picture, most soft tissue doesn't show up clearly. To
focus in on organs, or to examine the blood vessels that
make up the circulatory system, doctors must introduce
contrast media into the body.
Contrast media are liquids that absorb X-rays more
effectively than the surrounding tissue. To bring organs
in the digestive and endocrine systems into focus, a
patient will swallow a contrast media mixture, typically
a barium compound. If the doctors want to examine blood
vessels or other elements in the circulatory system,
they will inject contrast media into the patient's
bloodstream.
Contrast media are often used in conjunction with a
fluoroscope. In fluoroscopy, the X-rays pass
through the body onto a fluorescent screen, creating a
moving X-ray image. Doctors may use fluoroscopy to trace
the passage of contrast media through the body. Doctors
can also record the moving X-ray images on film or
video. |
Go to TOP
Are X-Rays Bad For You?
X-rays are a
wonderful addition to the world of medicine; they let doctors
peer inside a patient without any surgery at all. It's much
easier and safer to look at a broken bone using X-rays than it
is to open a patient up.
But X-rays can also be harmful. In the early days of X-ray
science, a lot of doctors would expose patients and themselves
to the beams for long periods of time. Eventually, doctors and
patients started developing radiation sickness, and the
medical community knew something was wrong.
The problem is that X-rays are a form of ionizing
radiation. When normal light hits an atom, it can't change
the atom in any significant way. But when an X-ray hits an
atom, it can knock electrons off the atom to create an
ion, an electrically-charged atom. Free electrons then
collide with other atoms to create more ions.
An ion's electrical charge can lead to unnatural chemical
reactions inside cells.
Among other things, the charge can break DNA
chains. A cell with a broken strand of DNA will either die or
the DNA will develop a mutation. If a lot of cells die, the
body can develop various diseases. If the DNA mutates, a cell
may become cancerous,
and this cancer may spread. If the mutation is in a sperm or
an egg cell, it may lead to birth defects. Because of all
these risks, doctors use X-rays sparingly today.
Even with these risks, X-ray scanning is still a safer
option than surgery. X-ray machines are an invaluable tool in
medicine, as well as an asset in security and scientific
research. They are truly one of the most useful inventions of
all time.